
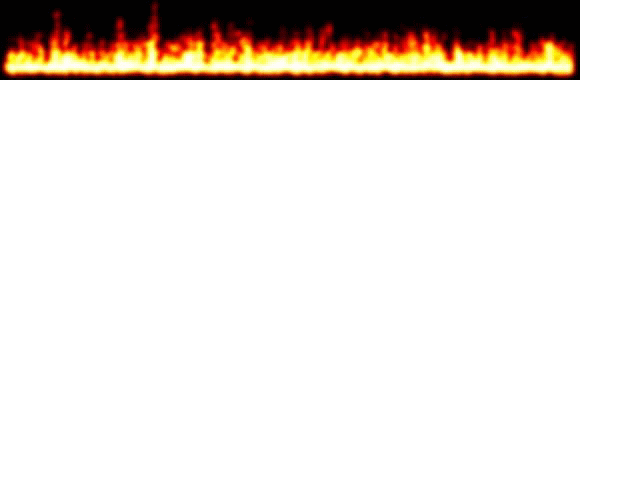
Volcanism & Volcanic Hazards
Summary of basic terms and concepts
William P. Leeman/Rice University
Global Volcanism
On any given day, there are at least several volcanoes active in various parts of the world. Current volcanic activity is conveniently summarized in Earth Alert (also published weekly in the Houston Chronicle, Monday's Business section).
An index map of currently active volcanoes shows a strong correspondence with specific tectonic areas (also see other WWW sites reporting current activity). Many of these volcanoes (and particularly those associated with subduction zones pose some kind of threat to the people living nearby, and some may have global impacts. This page aims to summarize basic volcanological characteristics and to highlight relations between volcanic activity, types of hazards, and simple hazard assessment.
A nice Volcano Learning Module presents a useful overview and QuickTime animations of some of the principles covered in this page.
Go back to top of page
Fundamental properties of magmas
The properties of magmas are closely related to their compositions, and these control their behavior, evolution, mode of eruption, and ultimately the type and degree of hazard that they may impose.
- Magma types - chemistry
- MAFIC: basalt (45-53% SiO2)
- SILICIC: high-silica = andesite (53-63% SiO2), dacite (63-67%), rhyolite (>67%)
- Temperatures: basalt (1050-1200°C) to felsic (800-1000°C)
- Viscosity (stiffness, resistance to flow) vs. fluidity - relation to magma chemistry, volatile (gas) content, crystal content; viscosity increases with increasing SiO2 and /or crystal content, and with decreasing temperature and/or volatile (water) content
- Ignoring cases where external water plays a significant role, recurrence intervals [R, in years] are approximately R<10 Hawaiian - Strombolian, R = 50-150 for Vulcanian, R = 100-200 for Peleean, and R>200 for Plinian/Krakatoan type eruptions (see below for eruption types)
Magma formation - melting
Magmas are basically molten rock, formed deep in the Earth - either in the crust or upper mantle where temperatures exceed the melting point of rocks in those regions. Where magmas form depends on the temperature gradient and the availability of constituents like water that can affect melting temperatures of those rocks. A wide variety of magmas can be produced, and these can be loosely categorized in terms of their silica (SiO2) content. Generally, magma type corresponds to the composition of source rocks that are partially melted. Relatively silica-rich crustal rocks typically melt to form siliceous magmas, whereas silica-poor mantle rocks generally produce low-silica magmas.
However, a spectrum of magma compositions can arise due to variations in source rock composition, degree of melting of those rocks, and the specific history of a magma as it ascends toward the Earth's surface. For example, magmas formed or stored at different depths can mix to produce intermediate hybrids. Or, magmas can undergo crystallization as they rise into cooler regions; because the minerals formed in this process generally have compositions distinct from the magma itself, their removal can cause a progressive shift in the composition of the remaining magma - usually toward more silica-rich variants. Most magmas erupt carrying a few percent of mineral fragments produced by this process.
Primary magma formation mechanisms:
- decompression melting - hot mantle ascends and partially melts due to pressure release
- melting due to addition of a 'fluxing agent' such as water (e.g., subduction zones)
- melting due to heating by hot intrusive magma, usually basalt (e.g., melting of continental crust)
Important magma evolution/modification mechanisms:
- magma mixing in conduits or 'magma chambers'
- magma contamination by partial melts or fragments of wallrocks
- crystal fractionation - removal of crystals formed during cooling (e.g., settling of dense ones, floating of less dense ones)
Eruption & ascent
What causes magmas to rise in the first place? Magmas almost always have lower density and higher specific volume than the rocks from which they form, or than most deep crustal and mantle rocks through which they may ascend. Thus, the main driving force behind magma ascent is buoyancy. This may be enhanced in some magmas by expansion of gases released during decompression. Key processes include:
- buoyancy (due to density contrast); contribution of bubble nucleation (due to outgassing as pressure decreases)
- explosivity - related to volatile contents and magma chemistry (hence, viscosity)
- fragmentation (pyroclastic ejecta or tephra): bombs (liquid, boulder-size blobs), blocks (angular solid boulders), scoria (cinder material, vesicular), lapilli (fragments 2-60 mm diameter), sands and ash (<0.1 mm) (volcaniclastic terminology)
Go back to top of page
Eruption type is a function of magma composition, viscosity, and gas content (all influenced by plate tectonic setting), recurrence time between eruptions, magma volume, replenishment of magma reservoir, strength of surrounding rocks, and access of external water.
Non-explosive:
- Flood lavas - basaltic, can be highly voluminous - Iceland, Columbia River Plateau and other flood basalt provinces, lunar mare (cf. large igneous provinces or 'LIPs')
- Hawaiian style - similar to flood type, but with some tephra and fast-moving fluid lavas, often channelized; these tend to form large shield-like cones
- Mid-ocean ridges (e.g., Juan de Fuca Ridge) - largely restricted to spreading center rifts as small cones and sheet flows
Explosive (in order of increasing energetics):
- Strombolian - bombs, molten ejecta, lavas --> symmetrical scoria cones, mafic compositions
- Vulcanian - ejecta blocks, pasty silicic lavas --> scoria cones & stratovolcanoes of tephra layers and ejecta deposits
- Surtseyan - hydrovolcanic, magma and water mixtures --> large clouds of fine pyroclastic dust, near vent rings of coarser ejecta
- Vesuvian/Plinian - very explosive, wide distribution of tephra, can lead to caldera collapse in large stratovolcanoes (Vesuvius, Pinatubo)
- Peleean - collapse of ash columns --> pyroclastic flows (nuees ardentes), debris avalanche deposits or ignimbrites (Mt. Pelee, Martinique)
- Bandaian - lateral explosion --> cyclone-like (up to 150 km/hr) base surge deposits (Mount St. Helens)
Volcano forms and processes are directly related to physical properties, compositions, and volumes (eruptive rates) of magmas, which in turn influence style of eruption.
Go back to top of page
Principal Volcanic Hazards
Define terms, describe each volcanic hazard and the resulting deposits, provide a good example of a volcanic event where each was a contributing factor:
Hazardous Volcanic Events
Review descriptions of the following volcanic catastrophes (cf. Abbott, 1999). What was the principal process in each case that caused great loss of lives and/or property (in the prehistoric examples, what would have been the most devastating processes)?
See other examples and a Table of casualties.
Go back to top of page
Scales of impact
Distances covered
- lavas - up to >100 km (depending on magma type, viscosity, volume ejected, topography, etc.)
- glowing avalanches (nuees ardentes) - up to >50 km (roughly 10-20 * height of volcano)
- ash/tephra - 100s to 1000s of km (depending on volume of ejecta and column height [proportional to thermal energy released], wind velocity and distribution)
- volcanic projectiles (stones, bombs) - up to 50-100 km (depending on muzzle velocity, size and density of particles, ejection angle, etc.)
- mudflows/lahars - up to 300 km (Cotopaxi volcano)
Areas covered per eruptive phase
- flood basalt eruptions - more than 10,000 km2 (but commonly 10s -100s); 1783 Laki eruptions covered 565 km2
- glowing avalanches - up to 100s km2 (1902 Mt. Pelee, >80 km2)
- ignimbrites (ash flow tuffs) - up to 1000s km2 (cf. U.S. examples)
- lahars (up to 1000s km2)
Volumes extruded
Volcanic Explosive Index [VEI] - roughly tenfold increase in ejecta volume (and energy release) for each VEI-step; applied to explosive or pyroclastic volcanoes. Certain types of eruptions (e.g., flood basalts, ignimbrite flows) are not included. Also, time-history of eruptive activity is highly variable between volcanoes - some deposits accumulated over significant time periods (years, Mazama) whereas others were formed in days (Krakatoa).
- 0 - only fumarolic activity
- I - <0.00001 km3 (e.g., 1949 Yakeyama, old detritus)
- II - <0.0001 km3 (e.g., 1926 Tokachidake, old detritus)
- III - <0.001 km3 (e.g., 1893 Asama, 0.0005 km3 fragmental ejects)
- IV - <0.01 km3
- V - <0.1 km3 (e.g., 1959 Oshima; 0.03 km3 lavas)
- VI - <1 km3 (e.g., 1911 Taal; 1707 Fuji; 1980 Mt. St. Helens, 0.5 km3 pyroclastics)
- VII - <10 km3 (e.g., 1888 Bandai; 1.2 km3 old detritus)
- VIII - <100 km3 (e.g., 1883 Krakatoa, 18 km3; 1470 B.C. Santorini, 30 km3; Mazama, >40 km3 pyroclastics)
- IX - >100 km3 (e.g., 1815 Tambora, 150 km3 pyroclastics)
Maximum volumes for a single event generally increase with silica content and vent size - up to 100s - 1000s km3 for large caldera-related silicic eruptions; can be significant for large basalt eruptions.
Caldera sizes - range up to 100 x 35 km (Toba), 70 x 40 km (Yellowstone). There is a complete spectrum to smaller sizes. Number of examples decreases with increasing size.
Go back to top of page
Probabilities of eruptions
Recurrence intervals [R] for eruptions increase with size of eruptions (as indicated by volcanic explosive index [VEI]). R is a function of volume of magma input, type of magma, type of reservoir, and strength of containing rocks, among other factors (tectonic activity, access of water to magma body, triggering earthquakes, etc.). Larger R generally implies higher probability of a violent eruption. See discussion of future Cascade eruptions.
Areal density of volcanoes in regions - problems involved in counting distinct volcanoes - is a parasitic cone a different volcano? (recall Mt. Shasta, which has numerous parasitic cones on the main cone; also, the main cone sits on eroded remnants of at least 2 older stratocones).
Individual volcanoes - estimates are based on relatively few events in a comparatively short period of observation; average recurrence intervals and uncertainties (one standard deviation) are as follows for selected volcanoes:
- Mauna Loa - since 1832, an eruption has occurred on average every 3.8 ± 3.0 yr
- Kilauea - since 1750, averaged an eruption every 3.2 ± 6 yr
- Hekla (Iceland) - since 930 AD, averaged an eruption every 54 ± 36 yr
- Vesuvius - averaged an eruption every 5.3 ± 4.8 yr - because the last eruption occurred in 1944, a new one seems overdue and may be more violent than usual. However, activity may be cyclic as there were quiescent gaps between 1872-1906 (34 yr) and 1906-1944 (38 yr).
- Mt. Pelee - since 1792, avg 364 ± 473 yr between eruptions (VEI = 3-4)
- Fuji - since 781 AD, avg 71 ± 113 yr between eruptions (last eruption was in 1707!)
- Mt. St. Helens - since 1500, avg 37 ± 58 yr between eruptions, more violent events are less frequent
Go back to top of page
Among the many active volcanoes in the world, some in proximity to population centers have been designated as Decade Volcanoes to promote more intensive monitoring and volcanological study. Another source of info on Earth's active volcanoes.
See basic modules concerning volcanic risk assessment and approaches to dealing with volcanic threats.
This section addresses methods of assessing volcanic activity, pending eruptions and risk analysis; combinations of these indicators work best. See the USGS publication on this subject.
- changes in fumarolic/hydrothermal activity
- geodetic measurements - ground tilt or extension due to movements of magma into shallow conduits
- seismicity - A-type: high frequency harmonic tremor (due to rapid movement of magma and 'magmafracting' to create new channels); B-type: lower frequency seismicity related to deformation of wallrocks; increasing levels of seismicity (#s of EQs) and ratio of (#B-type)/(#A-type) signify increased probability of eruption
- changes in levels of magmatic gas emissions (CO2, SO2, Cl, F) derived from rising, decompressing magma
Further WWW resources provide valuable information:
Other indicators
- prior eruptive history - recurrence intervals, cyclic behavior
- historic patterns of eruptions
- expected magma types, and their characteristics
Location, tectonic setting
- proximity to population centers & population distribution (compare hazards expected at Mt. St. Helens vs. Vesuvius - both of similar size and explosivity)
- proximity to coasts - tsunami risks to more distal regions
- geographic/topographic influences - channeling of volcanic deposits (see case studies for Mt. Ranier area)
Go back to top of page
Summaries of common magma types, and types and sizes of eruptions.
Study questions
Useful discussion and study and review questions can be found in the textbook Natural Disasters, P.L. Abbott (1999). You should also explore additional WWW resources, particularly Tilling et al..
To test your understanding of volcanic processes and hazards, try to develop appropriate hazard mitigation plans for various real world scenarios.
Related web pages:
Go back to top of page
LAST MODIFIED:
22 Feb 99
BY: Bill Leeman