Reviews
in Undergraduate Research - Issue 1
CP
Violation and the Dominance of Matter
}
James Morris
University of Arizona
Communicated By: Dr. Elliott Cheu
University of Arizona - Department of Physics |
SUMMARY
The observable Universe reveals a clear dominance of matter over antimatter.
If this asymmetry is consistent with the Universe as a whole, then a
contradiction arises between observation and any naïve formulation
of Big Bang theory. CP violation, violation of the combined C (charge
conjugation) and P (parity) operators, is one of three necessary conditions
of matter dominance theorized by Sakharov in 1967. Therefore, further
study of CP violation is warranted to determine its role in the evolution
of a matter-dominated Universe.
There are two ways by which violation might occur: direct and indirect.
The first possibility is that physical eigenstates may be written as
a linear combination of CP eigenstates - a small amount of the opposite
CP eigenstate might cause CP violation. This violation from mixing of
CP eigenstates is also called indirect CP violation. Independent of
indirect violation, CP violation might also occur in the decay or direct
CP violation. A famous experiment, which investigated the possibility
of indirect CP violation, was conducted in 1964. Since then several
experiments have been conducted to determine if CP violation also exists
in the decay. This review summarizes the methodology of two such experiments.
Despite the progress made in understanding the nature of CP violation,
the future of research of CP violation remains promising. Several proposed
experiments involve determining the degree of CP violation in much more
rare decays. I summarize one such future experiment of analyzing CP
violation in these decays.
Finally, due to the rare occurrence of CP violation, future investigations
will require high rate experiments with high efficiency detectors. To
this end we test the efficiency of one such detector which may be used
in such experiments. A high rate environment similar to that expected
in proposed CP violating experiments was used to examine the detector's
behavior. In particular, this detector was tested using electrons from
KL decays ( ).
The high efficiency of this detector in this high rate environment is
a testament to the future viability of this and similar devices in the
investigation of CP violation.
MATTER DOMINANCE
The Naïve Big
Bang
Big Bang Theory is widely accepted among the scientific community
due to its empirical merits. For this reason, scientists have begun
to establish particulars and study predictions of the theory. One
prediction is that the early Universe was devoid of matter, containing
only energy in the form of photons. As the Universe expanded, matter
was able to form through processes such as
(where g denotes a photon, p a
particle, and
an anti-particle). As can be seen, these processes produce equal numbers
of particles and anti-particles. Naïve formulations of the Big
Bang, which consider these processes alone, would result in a Universe
that is matter/antimatter symmetric. However, we observe matter -
not antimatter - out to 10Mpc (Dolgov, 1997), and strong arguments
can be made in extending this observation to the Universe as a whole.
Therefore, a contradiction arises between the small ratio of antimatter
to matter, which current estimates place at 10-4, and a
naïve formulation of the Big Bang.
Baryogenesis
The vast majority of matter
is comprised of particles called baryons. Baryons, such as
protons and neutrons, are particles with three quarks. There are two
ways by which the baryon (or matter) asymmetry in our universe can
be explained: either the abundance of baryons is the result of some
set of initial conditions or the abundance came about by some physical
mechanism. In 1967 Sakharov showed that at least three conditions
are required for such a mechanism to exist (Sakharov, 1967). The conditions
are
1. Baryon number violation
2. Thermodynamic Nonequilibrium
3. C and CP violation.
If some mechanism satisfies
these three conditions, the baryon dominance we see today may be created
from an early Universe which consisted mostly of energy. This is commonly
referred to as baryogenesis. This review will concentrate on the CP
violating condition of baryogenesis.
CP violation and
Baryogenesis
Quantum mechanically, CP violation implies that the system
is not symmetric under combined operations of charge conjugation
(C) and parity inversion (P). The operation of charge conjugation
exchanges particles with anti-particles, and the operation of parity
inversion changes the signs of the coordinates. The combined operation
of C and P relates the rates of particle transitions to anti-particle
transitions. If CP were conserved, then the rate for a particular
particle decay would be the same as that for the related anti-particle
decay. If CP is violated, these transition rates would differ. For
example, if the transition rate from anti-particle to particle is
greater than the transition rate from particle to anti-particle, then
matter creation would be preferred over antimatter creation.
NEUTRAL KAON AND
CP CONSERVATION
CP violation was first discovered in decays of the K meson (a meson
is a particle comprised of quark anti-quark pairs), also known as the
kaon. Kaons have the unique propriety of being produced by the strong
force and decaying via the weak force. This occurs because the kaon
is the lightest particle with a strange quark. So, in order for the
kaon to decay, its daughter particles cannot contain any strange quarks.
However, the strong force preserves quark type. So, the kaon cannot
decay via the strong force, but instead decays via the weak force, which
allows quark flavor to change. Due to this phenomenon, one can write
the weak eigenstates as superpositions of the strong eigenstates as
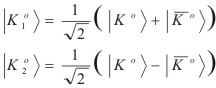
where  are
the weak eigenstates, and  the
strong eigenstates. Furthermore, CP changes into
and
vice versa. So, if we operate with CP on we
obtain an eigenvalue of +1, which is CP even. Similarly, if we operate
with CP on we
obtain an eigenvalue of -1, which is CP odd. If CP is conserved, the
weak eigenstates K1 and K2 above are identically
the CP even and CP odd states, respectively
Because the K1 and the K2 are distinct under CP,
their decay products must also be distinct. The K1 decays
primarily into a state with two pi mesons (pions), which is even under
CP, while the K2 decays primarily into a state with three
pi mesons, which is odd under CP. The three pion state has a mass very
close to the kaon. Because of this, there is very little extra energy,
or phase space, available for the decay to occur. This causes the K2
to decay much less readily than the K1, which has significantly
more phase space. In fact, the K2 has a lifetime approximately
600 times greater than the K1. For this reason the K1
is usually called KS and the K2 is called KL,
where S and L designate the short and long lifetimes.
As mentioned, if CP is conserved, the KS should be equivalent
to the K1 state and KL should be equivalent to
the K2 state. Furthermore, if CP is conserved we expect that
the K1 (or KS) should decay only into states such
as KS ® 2p,
and the K2 (or KL) should decay only into states
such as KL ® 3p.
CRONIN-FITCH EXPERIMENT
AND INDIRECT CP VIOLATION
An obvious method to determine
if CP is conserved is to produce a well-defined CP state and observe
its decays. If the initial state has different CP than the final state,
then CP has been violated. In 1964 Cronin et al. performed this type
of experiment (Christenson et al., 1964). They produced a beam of K
mesons, which was allowed to travel a relatively long distance. The
region near the end of the beam line contained mostly K2
mesons, K1 mesons having decayed away, so they expected to
only see decays from K2 ®
3p. A signal for CP violation would
be the appearance of K2 ®
2p decays in their detector.
The group used the Brookhaven AGS (Alternating Gradient Synchrotron)
particle accelerator. A beam of protons bombarded a Be target producing
an assortment of particles. Large magnets called sweeping magnets
were used to remove charged particles so that a neutral kaon beam
was produced. As the kaons proceeded down the beam line, they decayed,
and the resulting daughter particles were detected by two detectors
located at the end of the beam line. These detectors measured the momentum
and positions of the daughter particles, which could be used to determine
the momentum vector of the parent kaon particle. Because the detector
only reconstructed two of the three daughter particles, the momentum
vector of 3p decays reconstructs
to a non-zero angle with respect to the original K2 beam.
On the other hand, the momentum vector of 2p
decays reconstructs coincident with the original K2 beam.
A sophisticated computer program was used to determine the expected
results of this angle if CP were conserved. This Monte Carlo simulation
was compared to the results recorded from the experiment. Figure 1 shows
the distribution of the angles between the reconstructed momentum vector
and original K2 beam for all decays in a given mass region.
Notice the significant peak above the Monte Carlo expectation, corresponding
to zero angle decays. After subtracting backgrounds the group measured
45 ± 10 K2 ®p+
+ p- decays of the 22,700
K2 decays - the first evidence of CP violation.
|
Figure
1. Angular
distribution of events with mass 490 < m* < 510 MeV |
Indirect CP violation
The Cronin experiment demonstrated that CP is violated in
weak decays. This discovery lead to the realization that the weak
eigenstates are not the same as the CP eigenstates. In fact, one can
write the weak eigenstates as admixtures of the CP eigenstates
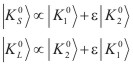
In order for KL
and Ks to be pure CP eigenstates the mixing parameter must go to zero,
conserving CP. However, the Cronin experiment demonstrates that e
must be nonzero, so the weak eigenstates are not pure eigenstates
of CP but contain a small amount of the "wrong" CP value.
For example, KS is not purely CP even but contains a small
amount, e, of CP odd. Furthermore,
we notice that e is independent
of decay channel so we expect CP violating effects to have the same
rate whether the decay mode is KL ®p°
+ p° or KL ®p+
+ p-. Because this
violation is due to mixed eigenstates, it is referred to as CP violations
through mixing or indirect CP violation. The Cronin experiment
obtained a parameter value of e
» 2.3 X 10-3
which corresponds to 1 violation for every 500 decays.
DIRECT CP VIOLATION,
NA48, AND KTeV
Direct CP Violation
The Cronin
experiment showed that CP violation occurs when a state is composed
of mixed CP eigenstates. The K®pp
decay occurs through the K1 component. CP violation however,
might occur in the direct decay of either K1 or K2,
so that the K2 component might simply decay into two pions.
This is called direct CP violation, the degree of which is
measured by a parameter e'. Note
that this violation does not appeal to an admixture of CP states but
occurs directly in the decay. This is why direct CP violations are
sometimes referred to as violations in the decay. So there
are two means by which CP may be violated. Either the K1
component of the KL decays into two pions (indirect CP
violation). Or, the KL decays into two pions via the K2
state (direct CP violation). These possibilities are shown below:
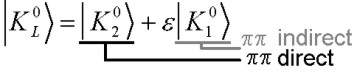
e'/e
and
the Double Ratio
Although indirect
CP violation manifests itself in the KL ®2p°
decay, CP violation also occurs in other decay modes such as KL
®p+
+ p-. If all CP violating
effects were due to indirect CP violation, which is decay channel
independent, then the rates for different decay modes would be the
same. So if one were to compare the indirect violation in two different
modes, and find different rates, this must be due to a direct CP violation.
One method to search for direct CP violation is to compare K ®p°p°
decays to K®p+
+ p- decays. The ratio
e'/e
can be determined from the following ratio
of CP violating rates:
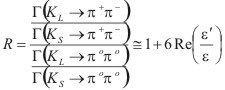
where G
designates the rate of occurrence of the decay (Alavi-Harati, 1999).
Notice that the numerator and denominator of this ratio are both measures
of indirect CP violation, where we expect the KL violations
to occur about once in every 500 decays. If direct CP violation does
not exist, we would expect the double ratio to equal unity, forcing
the e'/e
term to vanish as expected. However, if CP
violations are decay channel dependent, the ratio R
will deviate from unity resulting in a nonzero e'/e
, evidence of direct CP violation.
NA48 and KTeV
Searches for direct CP violation
have been performed since the Cronin experiment, but it was not until
the last decade that experiments have obtained statistically significant
results of direct violation. The difficulty in determining e'/e
is due to the fact that direct CP violation
is expected to be much smaller than indirect CP violation. About five
years ago two promising experiments set out with a goal of obtaining
a definitive value of e'/e.
The determination of the double ratio amounts to essentially a counting
experiment. The two CP violating rates are just proportional to the
number of particles from each decay mode within a certain decay volume.
The difficulty in this measurement lies in efficiently determining
the decay rates while subtracting errors from backgrounds and accidentals.
One advantage of this measurement is that a number of systematic effects
will cancel in the double ratio. So, it is important to collect all
four decay modes simultaneously. As mentioned, the Cronin experiment
recorded about 22,000 events of which 45 ± 9 were indirect
CP violating. However, to measure e'/e
to the desired precision nearly 20 million
indirect CP violating decays have to be collected (Alavi-Harati, 1999).
In 1997, a group in experiment NA48 at CERN took data used to analyze
e'/e.
In three separate runs that lasted months at a time, NA48 was able
to record over ten million events per run. This large number of events
is possible due to the high rates produced by today's particle accelerators
and the high efficiency of modern particle detectors. NA48 utilized
a liquid krypton calorimeter to measure energy deposition and a bent
crystal to extract protons onto a KS target. Such a setup
is necessary to produce a simultaneous collinear KS beam,
which is required to eliminate errors in the double ratio. After three
years of taking data, NA48 reported a value of (15.3 ± 2.6)
X10-4 for e'/e
(Lai, 2001).
A second group under the KTeV collaboration used the Tevatron at Fermilab
in investigation of e'/e.
The setup and general methods are similar with NA48, but various components
are constructed differently. For example, KTeV uses a pure CsI crystal
calorimeter to measure energy deposition. To create the collinear
KS beam, KTeV uses a unique property of kaons whereby a
KL turns into a KS when passed through a thin
slab of matter. This is due to the strong interactions of kaons with
protons and neutrons when passing through matter. (This regeneration
process was also studied in the Cronin experiment.) Every second,
200 billion protons hit a beryllium target similar to the one used
in the Cronin experiment (Cheu, 1997). An extremely high rate trigger
determines 53 million times a second which events to use in data analysis.
This corresponds to 10 MB of data every second, which over the KTeV
running period, results on the order of 100 TB of data collected.
After analyzing only one eighth of their data, the KTeV group was
able to attain a statistically significant result much larger than
the theoretical expectations of (4-10) X 10-4 (Ellis, 1976
and Ciuchini,1997). Combined with later data, which amounts to about
one-half of the total KTeV data, resulted in a value for Re (e'/e)
of (20.7 ± 2.8) X 10-4.
Overall Average
After averaging all
recent direct CP violation experiments, one obtains a world average
of (17.2 ± 1.8) X 10-4 as shown in Figure 2. This
result conclusively shows that direct CP violation exists, a new form
of matter/antimatter asymmetry. Note that the small value of this
result signifies that direct CP violation is much smaller than indirect
violation.
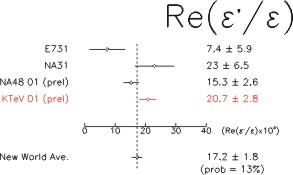 |
Figure 2. Values
reported by various experiments for e'/e and the world average. |
THE FUTURE OF CP
VIOLATION
KOPIO
The KTeV and NA48 experiments combined with the Cronin experiment
definitively show that there are two types of CP violation: direct
and indirect. However, theoretical calculations of e'/e
are very challenging because of our lack of
understanding of the strong force. To achieve a better understanding
of CP violation, an investigation of other decay channels is necessary.
The KOPIO experiment using the Brookhaven AGS - the same used by Cronin
et al. - will attempt to study one of the more rare decay channels.
In particular, the decay
has some interesting features which are worthy of investigation. In
this decay the amplitude for the indirect CP violating component is
actually expected to be much less than the amplitude for the direct
component. In addition, the theoretical uncertainties for this decay
mode are very small because the final state does not include any hadronic
particles such as pions, which are theoretically difficult to handle
because they involve the strong interaction. So, measurement of this
decay mode would allow a much better understanding of direct CP violation
(Littenberg, 1989). This decay occurs about once in every 100 billion
decays, making it particularly difficult to study. Experimentally,
the final state consisting of two photons (from the p°)
and two neutrinos is difficult to reconstruct. Because the neutrinos
only interact via the weak interaction, they will remain undetected
in the experimental apparatus, so the challenge is locating two isolated
photons and nothing else in the detector. Given the very high rate
of backgrounds expected in this experiment, the KOPIO group faces
a number of challenging tests in searching for CP violation in rare
decays. However, a positive result from this group will help to further
our understanding of CP violating effects.
New Detectors
The next generation of particle
detectors and the next batch of CP violation experiments will continue
to push the limits of our current technology. More than likely, the
next generation of experiments will require much more precise and
accurate detectors with the ability to obtain high precision under
extreme conditions. One such detector has been examined under the
KTeV project at the University of Arizona.
This detector is composed of alternating bars of quartz and uranium,
and is situated directly in the beam line. This is a unique region
for detectors, which are usually adjacent to the beam line where the
interaction rate is much lower. A detector residing in the beam line
is well-suited for experiments like KOPIO, where there are many background
processes to a decay such as .
Such backgrounds can fake a signal if some of the daughter particles
continue down the beam line and remain undetected. Placing such a
detector in the beam line will reduce the probability for such backgrounds.
The quartz-uranium detector operates by measuring the scintillation
light resulting from interactions of charged particles with the quartz
bars. Photons also can be detected as they shower in the heavier uranium,
producing a multitude of charged and neutral particles. The measure
of the resulting scintillation light is proportional to the amount
of energy deposited.
In analyzing the detector's efficiency, KL decays were
reconstructed in the KTeV detector. Since the environment in the KTeV
detector is similar to that expected in future experiments, this analysis
should indicate how our quartz-uranium detector will perform in an
experiment such as KOPIO. The electrons from KL decays
are identified using the KTeV detector, which is upstream of our quartz-uranium
detector. Then, with the particles' identified, we test the ability
of the quartz-uranium detector to correctly identify them. Despite
the high rate environment of over 1 MHz, the detector is still able
to properly identify particles correctly with very high efficiency
(in this case electrons). In the KOPIO experiment, one is more interested
in photons, but since electron and photon interactions are expected
to be similar in our detector, these results indicate how well our
quartz-uranium detector should perform for photons. Our result is
no misidentified electrons from more than 40,000 events used in analysis.
This places an upper limit of the detector's inefficiency at 5.0 X
10-5 at the 90% confidence level. This high efficiency
signifies a promising future for tomorrow's detectors in future experiments
such as KOPIO.
CONCLUSIONS
CP Violation Today
Although indirect CP violation
was first discovered by Cronin et al. in 1964, the search for direct
CP violation is a fairly recent endeavor. It was only in the last
decade that a high enough precision has been reached to verify direct
CP violation. Direct CP violation has been discovered and, as expected,
it has a much smaller effect than indirect violation. Furthermore,
CP violation is still in the early stages of understanding. We await
experiments such as KOPIO to obtain a new outlook on direct CP violation.
An interesting possibility in these experiments is the discovery of
physics beyond the standard model. The standard model is the set of
current theoretical facts, confirmed from experiment, which govern
particle physics. The standard model is able to make certain theoretical
predictions which experiment will confirm or deny. Thus far the standard
model is consistent with experiment; however, if an inconsistency
is discovered this will give rise to go beyond current physics and
establish a new theory. CP violation is a phenomenon which may provide
this avenue away from the standard model.
Baryogenesis Revisited
Over the past three decades
a preference of over
has been established through CP violation. Thus one of the Sakarov
conditions is now known to exist. Note however, that although CP violation
exists, CP violation in weak decays cannot account for the degree
of matter/antimatter asymmetry in the Universe. In fact CP violation
in weak decays is too small by a large factor to account for baryogenesis.
Future experiments will search for CP violation elsewhere, which is
believed to manifest itself B meson system also; however current theory
points to no resolution even under this consideration. To resolve
this issue we might need to appeal to CP violation in models currently
beyond the standard model and outside of the realm of current experimental
confirmation. It may be that only under considerations of Supersymmetric
models (SUSY) or models at the level of Grand Unified Theories (GUT)
will the riddle of matter dominance finally be solved.
ABOUT THE AUTHOR
James Morris is an
undergraduate senior attending the University of Arizona. He plans on
attending a doctorate program in physics. He aspires to become a professor
of physics at the university level and to contribute to current research
in the field. James assists the high energy department at the University
of Arizona. In particular he is testing the efficiency of next-generation
particle detectors for KTeV which may be used in future experiments
involving CP violation. The detector under analysis is a quartz-uranium
detector which has the uncommon feature of residing directly in the
beam line. Such a region is rare for particle detectors, which usually
reside adjacent to the beam line in detecting electromagnetic showers.
This region exposes the detector to a high rate environment on the order
of 1 MHz. And this fact combined with the higher precision required
by future experiments creates the need for a high efficiency. Despite
these circumstances the detector is still able to attain high efficiency.
ACKNOWLEDGEMENTS
I would like to thank
the High Energy group at the University of Arizona for their assistance
and use of their resources. I am particularly grateful to Dr. Elliott
Cheu for his assistance and guidance on this project.
FURTHER READING
Baym, G. (1973). Lectures
on Quantum Mechanics. (Menlo Park, CA: Benjamin/Cummings
Publishing).
Bigi, I.I., and Sanda, A.
I. (2000). CP Violation. (New York, NY: Cambridge University Press).
Branco, G. C., Lavoura, L.,
and Silva, P.J. (1999). CP Violation. (New York, NY: Oxford University
Press).
Cahn, R.N., and Goldhaber,
G. (1995). The Experimental Foundations of Particle Physics. (New York,
NY: Cambridge University Press).
REFERENCES
Alavi-Harati, A., et al.
(1999). Observation of direct CP Violation In K(S,L) --> PI PI Decays.
Phys. Rev. Lett. 83, 22.
Cheu, E., et al. (1997).
Proposal to continue the study of direct CP violation and rare decay
processes in KTeV in 1999. FERMILAB-P-0799
Christenson, J.H., et al.
(1964). Evidence for the 2p decay of the K2o meson. Physical
Review Letters. 13, 138-140.
Cicuchini, M. (1997). Epsilon-Prime
/ Epsilon: Three Years Later. Nucl.Phys.Proc.Suppl. 59.
149-156.
Dolgov, A.D. (1997). Baryogenesis,
30 Years After. Presented at 25th ITEP Winter School of Physics TAC-1997-024.
[HEP-PH 9707419]
Ellis, J., Gaillard, M. K.,
and Nanopoulos, D. V. (1976). Lefthanded Currents And CP Violation.
Nucl.Phys. B109; Gilman, F. J. and Wise M. B. The Delta I = 1/2 Rule
and Violation of CP in the Six Quark Model.Phys. Rev. Lett. B83.
83.
Lai, A. (2001). A Precise
Measurement of the Direct CP Violation Parameter RE (Epsilon-Prime /
Epsilon). Eur. Phys. J. C22. 231-254.
Littenberg L. (1989) The
CP Violating Decay K0(L) ---> PI0 Neutrino Anti-Neutrino. Phys.
Rev. D39. 3322-3324
Longair, M. S. (1998). Galaxy
Formation. (Heidelberg, Berlin: Springer) pp. 231
Sakharov, A.D. (1967). Violation
of CP Invariance, C Asymmetry, and Baryon Asymmetry of the Universe.
Pisma Zh.Eksp.Teor.Fiz. 5, 32-35.
Steigman, G. (1976). Observational
tests of antimatter cosmologies. Annual review of astronomy and astrophysics.
14, 339-372.
|